Encourager la durabilité avec la production d'ammoniac vert
L'ammoniac est un composant essentiel des engrais et de nombreux produits de consommation quotidiens. Alors que l'industrie poursuit des initiatives pour atteindre le zéro net émission, l'ammoniac vert offre un potentiel de réduction du carbone, mais plusieurs défis restent à surmonter avant sa production à grande échelle
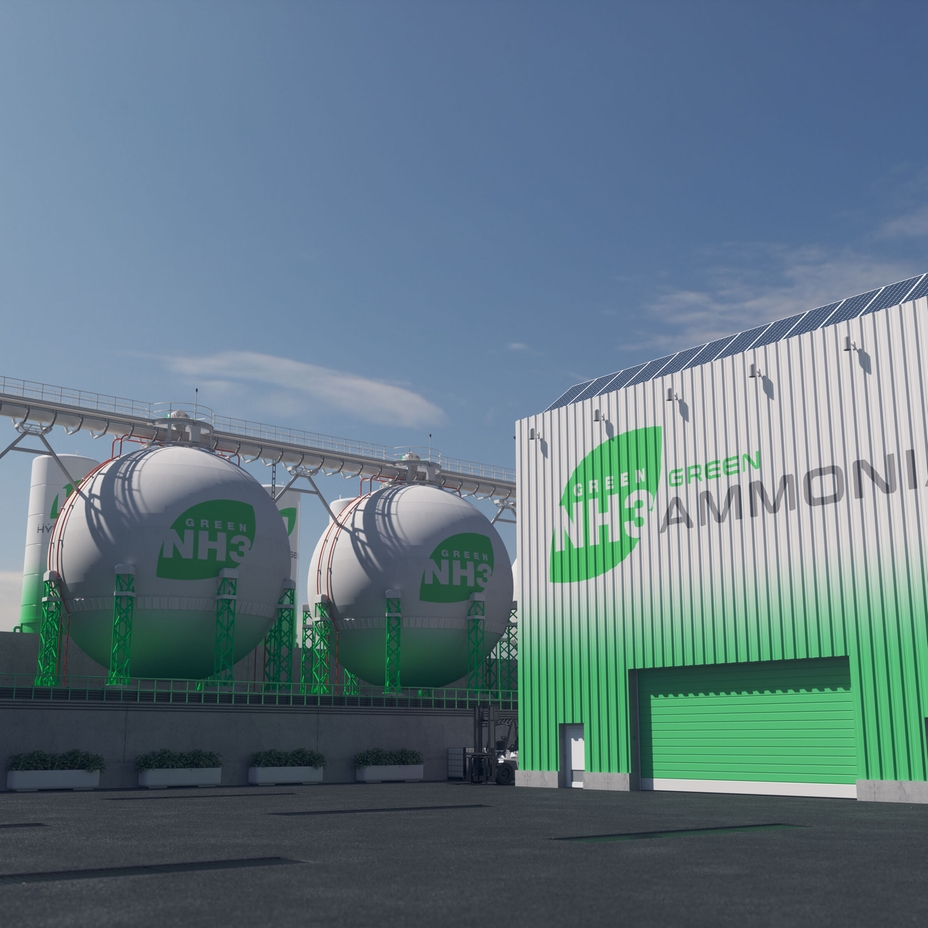
En bref
- Utilisé dans la production d'engrais, les produits pharmaceutiques, les systèmes HVAC et plusieurs autres industries, l'ammoniac est essentiel pour la population mondiale et divers aspects de la société moderne.
- L'ammoniac est produit principalement à l'aide du procédé Haber-Bosch, basé sur l'hydrogène dérivé des combustibles fossiles et qui contribue aux émissions de gaz à effet de serre. De plus, le process lui-même est alimenté par des sources d'énergie fossiles.
- L'azote est l'autre composant essentiel de la production d'ammoniac et les méthodes de production classiques ont également besoin d'énergie dérivée de combustibles fossiles.
- L'utilisation de sources d'énergie renouvelables telles que le soleil, le vent et l'eau pour la production d'hydrogène vert par électrolyse de l'eau et autres process permettent d'obtenir de l'ammoniac vert, avec une empreinte carbone moindre.
- Les coûts initiaux plus élevés pour les infrastructures d'énergie renouvelable et les électrolyseurs entravent actuellement l'adoption de l'ammoniac vert. Toutefois, à mesure que ces technologies évoluent, les coûts devraient diminuer, ce qui rendrait l'ammoniac vert plus compétitif.
- L'ammoniac exige des protocoles de sécurité rigoureux, comprenant des systèmes de détection des fuites et des procédures pour une manipulation sûre. L'instrumentation avancée joue un rôle essentiel dans la surveillance et l'optimisation des process de production pour améliorer l'efficacité et la sécurité.
Un produit chimique polyvalent pour l'industrie
L'ammoniac est un composé d'azote et d'hydrogène et est vital pour de nombreuses applications quotidiennes du monde moderne. Plus important encore, il joue un rôle essentiel dans la production de produits alimentaires en tant que source d'azote primaire pour les engrais, afin d'assurer une agriculture suffisante pour nourrir la population mondiale en pleine croissance.
Au-delà de l'agriculture, l'ammoniac est largement utilisé dans diverses applications industrielles, notamment :
- La production chimique, en fournissant une base pour divers produits, y compris les matières plastiques, les fibres, les colorants et les explosifs.
- La fabrication pharmaceutique, comme matière première pour divers ingrédients pharmaceutiques actifs et pour contrôler le pH.
- Les systèmes HVAC, pour les fluides frigorigènes, grâce à ses propriétés efficaces du transfert de chaleur.
- L'industrie agro-alimentaire en tant qu'additif et conservateur.
- Le traitement de l'eau, pour purifier les approvisionnements en eau en créant des chloramines, ce qui facilite la désinfection organique.
- Les produits de nettoyage, comme ingrédient clé de nombreux agents domestiques grâce à ses propriétés de dégraissage et de désinfection.
- La fabrication de textiles, pendant le processus de finition pour améliorer la souplesse du tissu et l'affinité des colorants.
Alors que l'ammoniac est un produit chimique inestimable dans de nombreuses industries, les méthodes classiques de production d'ammoniac ont des impacts environnementaux, ce qui pousse à rechercher des solutions alternatives plus vertes.
Production d'ammoniac classique avec Haber-Bosch
L'ammoniac est principalement produit à l'aide du procédé Haber-Bosch, où l'azote est combiné à l'hydrogène à pression et température élevées en présence d'un catalyseur.
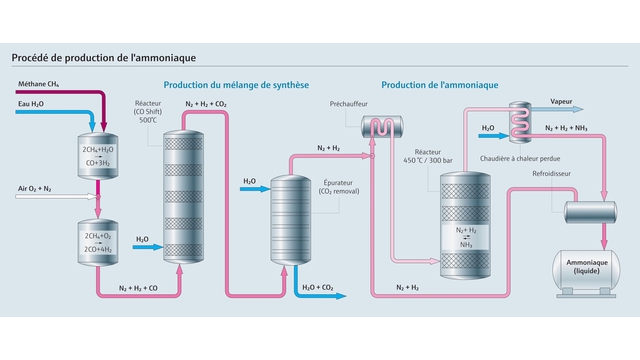
Les étapes comprennent :
- Extraction d'azote : l'azote N2(N2) est extrait de l'air à l'aide d'une unité de séparation de l'air à travers un procédé de liquéfaction et de distillation. Une autre possibilité est l'adsorption à variation de pression, qui fait appel à des matériaux adsorbants spéciaux pour capter de manière sélective des molécules d'azote.
- Production d'hydrogène : L'hydrogène (H2) est généralement obtenu par reformage du méthane à la vapeur ou par reformage autothermique.
- Synthèse de l'ammoniac : les gaz d'azote et d'hydrogène purifiés sont mélangés et passés dans un catalyseur - généralement de fer - à haute pression (> 200 atm/2 940 psi) et haute température (> 450 °C/842 °F), ce qui crée de l'azote et de l'hydrogène élémentaires, combinés pour former de l'ammoniac (NH3).
- Refroidissement de l'ammoniac de l'azote et de l'hydrogène résiduels : Les gaz quittent le réacteur à une température de > 450 °C/842 °F et sont refroidis par un échangeur thermique doublé d'une chaudière de récupération. Cela produit de la vapeur surchauffée, qui est ensuite utilisée dans une unité de production d'énergie.
- Séparation de l'ammoniac : le mélange gazeux est refroidi à environ -40 °C (-40 °F), ce qui provoque la condensation de l'ammoniac afin qu'il puisse être séparé, grâce à son point d'ébullition plus élevé, des gaz d'azote et d'hydrogène n'ayant pas réagi. Ces gaz n'ayant pas réagi sont recyclés dans le process pour terminer leur réaction.
Le processus global est représenté ainsi : N₂ + 3 H₂ ↔ 2NH3
La méthode Haber-Bosch est fortement énergivore et les processus de production d'hydrogène marron, noir et gris contribuent aux émissions de gaz à effet de serre (GES). Cet impact environnemental entraîne une préférence pour l'hydrogène vert provenant de sources durables et pour la production d'azote avec des énergies renouvelables.
Production d'ammoniac vert
La production d'hydrogène utilisée pour créer l'ammoniac représente environ 1,8 % des émissions mondiales de dioxyde de carbone, un chiffre comparable à l'ensemble de l'industrie aéronautique. En réponse à ces préoccupations environnementales, le concept de « l'ammoniac vert » vise à réduire considérablement l'empreinte carbone de la production d'ammoniac en assurant l'approvisionnant en composants gazeux par le biais de process à zéro émission nette, entraînés par des sources d'énergies renouvelables.
Aperçu
La production d'hydrogène utilisée pour créer l'ammoniac représente environ 1,8 % des émissions mondiales de dioxyde de carbone, un chiffre comparable à l'ensemble de l'industrie aéronautique.
Les matières premières pour la production d'ammoniac vert correspondent à trois besoins simples : l'air, l'eau et l'énergie renouvelable pour alimenter les différents process.
À l'aide de la distillation cryogénique ou de l'adsorption à variation de pression, les unités de séparation de l'air extraient l'azote de l'atmosphère. La distillation cryogénique fonctionne en refroidissant l'air à des températures extrêmement basses, séparant ses composants gazeux - y compris l'azote nécessaire à la production d'ammoniac - du fait de leur points d'ébullition différents. Une autre possibilité est l'adsorption à variation de pression, qui fait appel à des matériaux adsorbants spéciaux pour capter de manière sélective des molécules d'azote.
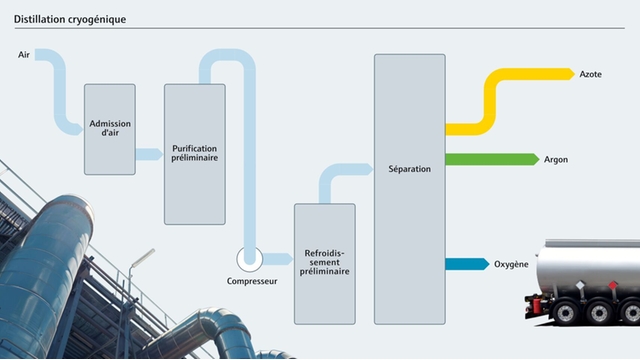
L'ammoniac vert se distingue avant tout par l'utilisation d'hydrogène vert, produit par l'électrolyse de l'eau, process lui-même alimenté par des énergies renouvelables, qui crée zéro émission nette. Ce process consiste à fractionner les molécules d'eau dans leurs atomes d'hydrogène et d'oxygène ; l'hydrogène est alors récolté comme matière première pour la synthèse de l'ammoniac et l'oxygène, inoffensif, est rejeté dans l'atmosphère. L'électrolyse est réalisée principalement à l'aide d'électrolyseurs alcalins, une technologie mature et éprouvée, ou d'électrolyseurs à membrane échangeuse de protons (PEM), connus pour une plus grande efficacité et des temps de réponse plus rapides, mais plus onéreux.
L'azote et l'hydrogène sont ensuite mis à réagir selon le procédé de Haber-Bosch, mais avec une empreinte carbone réduite. L'ensemble des process doivent être alimentés par des énergies renouvelables pour que l'ammoniac qui en résulte puisse être considéré « vert ».
L'ammoniac peut également être décomposé en molécules d'hydrogène par le process du craquage de l'ammoniac, mais cette réaction demande une énergie considérable, nécessitant une chaleur de 600 °C/1 112 °F en présence d'un catalyseur. Ce process est utilisé pour certaines applications qui nécessitent de l'hydrogène parce que l'ammoniac est plus facile à manipuler et stocker en vrac que l'hydrogène pur.
Surmonter les défis économiques
Comme pour beaucoup de process à faibles émissions de carbone, la mise à l'échelle de la production d'ammoniac vert est confrontée à des difficultés en termes d'infrastructures et de coûts de production. Tout d'abord, le secteur des énergies renouvelables, même s'il évolue rapidement, n'a pas encore atteint le niveau d'intégration réseau et de stockage des systèmes conventionnels, ce qui est nécessaire pour répondre aux demandes des industriels et consommateurs. De même, la mise à niveau des électrolyseurs nécessaires à la production d'hydrogène vert implique encore des investissements importants.
Toutefois, à mesure que les technologies durables évoluent et que les économies d'échelle sont réalisées, la production d'ammoniac vert va devenir de plus en plus compétitive. Le coût des énergies renouvelables est sur le déclin ces dernières années et cette tendance devrait se poursuivre, ce qui rendra l'hydrogène et l'azote verts plus accessibles économiquement.
L'ammoniac est également à l'essai comme vecteur énergétique exempt de carbone - et même pour la combustion directe dans certaines applications
- ce qui aiderait à résoudre les problèmes d'intermittence des énergies solaire et éolienne. En outre, sa haute densité énergétique a conduit à des recherches pour l'utiliser comme carburant pour le transport maritime et long-courrier, des applications lourdes en carbone.
Réflexions sur la sécurité et instrumentation de pointe
Indépendamment de sa valeur, l'ammoniac est une substance chimique dangereuse et doit être manipulée avec prudence. L'inhalation de seulement 0,5 % d'air concentré en ammoniac peut être mortelle pour l'humain. Sa nature hautement corrosive et toxique exige des protocoles de sécurité rigoureux pendant la production, le transport et l'utilisation.
Outre l'équipement de protection individuelle standard et les formations globales des employés, les systèmes de détection des fuites aident à atténuer le danger en cas de rupture inattendue de l'enceinte. Ces systèmes sont composés de détecteurs de gaz électrochimiques, de transmetteurs de pression et d'autres composants et instruments de sécurité avancés.
De plus, les unités de séparation de l'air utilisées pour extraire l'azote de l'air pour la production d'ammoniac dépendent d'instruments comme les analyseurs TDLAS pour l'analyse de la pureté et les capteurs de pression pour le contrôle du process. Dans le process de l'électrolyse de l'eau, les capteurs de conductivité surveillent la qualité de l'électrolyte, garantissant une performance et une longévité optimales de l'électrolyseur, tandis que les débitmètres aident à réguler la production d'hydrogène et d'oxygène.
La boucle de synthèse de l'ammoniac repose sur une large gamme d'instruments, y compris les capteurs de température, les transmetteurs de pression et les analyseurs de gaz, pour optimiser les conditions de réaction et assurer la qualité du produit. Ces instruments fournissent des données continues pour le contrôle en temps réel et l'analyse de l'historique, ce qui permet au personnel d'optimiser le process et de maximiser l'efficacité tout en maintenant des conditions d'exploitation sûres.
La décomposition de l'ammoniac exige des débitmètres massiques pour une livraison précise du gaz et des taux de réaction optimaux, des thermocouples pour une mesure précise de la température et des analyseurs de gaz pour la surveillance de la composition de la vapeur d'hydrogène pour assurer la pureté.
Perspectives vertes
Alors que l'ammoniac est incroyablement précieux dans de nombreux process industriels que les consommateurs considèrent souvent comme acquis, la généralisation de l'ammoniac vert fait toujours face à des obstacles. Les énergies renouvelables et les infrastructures d'électrolyse restent limitées et les coûts d'investissement sont élevés. En outre, le transport et le stockage sûrs de l'ammoniac nécessitent une attention particulière en raison de sa nature dangereuse, bien que les méthodes approuvées soient bien établies.
Le principal avantage de l'ammoniac vert est la dissociation de la production d'ammoniac des combustibles fossiles pour réduire les émissions de GES. En conséquence, il a attiré l'attention des gouvernements et de l'industrie et ces acteurs l'intègrent de manière stratégique dans les plans de durabilité. Il fournit une voie pour réduire l'empreinte carbone de l'industrie de l'engrais et d'une myriade d'autres secteurs qui dépendent fortement de l'ammoniac.
La poursuite des recherches et du développement, ainsi que l'innovation continue dans les technologies de production et d'utilisation, seront essentielles à la généralisation de l'ammoniac vert, dans un contexte où le monde cherche à atteindre le zéro net émission d'ici le milieu du siècle.